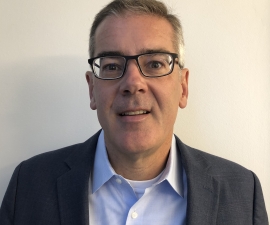
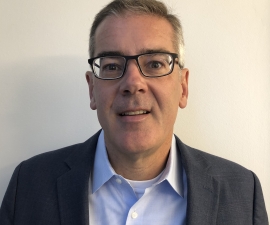
Research Expertise and Interest
inorganic and materials chemistry, synthesis of inorganic molecules and higher dimensional solids, precise tailoring of chemical and physical properties, gas storage, molecular separations and catalysis in porous materials, magnetic and conductive materials
Research Description
Research in the Long Group is focused on controlling chemical structure and function through molecular design. Within this paradigm, they employ the principles of coordination chemistry in the tailored synthesis of atomically precise molecules and materials with programmed properties, with the goal of achieving fundamental advances relevant to our technological, environmental, and energy future. They are developing new porous materials for diverse applications ranging from gas storage to water purification. They are also creating new high-performance magnetic molecules and conductive extended solids. They employ a range of physical methods to analyze and characterize their materials comprehensively, including gas adsorption analysis, x-ray and neutron diffraction, various spectroscopic techniques, and SQUID magnetometry. Current focus areas are highlighted below. For more information and a full list of publications, please visit the group website.
Functional Metal–Organic Frameworks
Metal–organic frameworks (MOFs) are crystalline, permanently porous solids with internal pore structures and local chemistries that can be precisely tuned using coordination chemistry. Guided by insights from molecular chemistry, biology, and traditional solid-state chemistry, they are harnessing the unique properties of MOFs (i) to achieve new mechanisms for cooperative gas adsorption reminiscent of those employed by natural enzymes; (ii) to design new porous magnets and electronic conductors; (iii) for applications in catalysis; and (iv) featuring coordinatively unsaturated metal sites and unique pore architectures for selective guest adsorption relevant to gas storage and molecular separations. They are also developing new methods to produce these adsorbents in predictable nanocrystalline forms to optimize macroscopic properties relevant for industrial applications, such as crystallite size and morphology, and incorporating these nanocrystals within polymer membranes to generate mixed-matrix membranes that exhibit exceptional selectivities and permeabilities for industrially relevant separations.
Quantum Magnetism in Molecular Materials
They are employing novel design principles and architectures precisely chosen to enhance operating temperatures within a class of compounds known as single-molecule magnets. These molecules exhibit a strong directional dependence to their magnetization (known as magnetic anisotropy) and magnetic hysteresis, previously thought to be exclusive to bulk magnets. As such, single-molecule magnets are of great interest for applications including quantum computing, quantum sensing, and even dark matter detection. Through the judicious manipulation of ligand fields, electronic structure, and magnetic interactions, they are endeavoring to create systems exhibiting striking new magnetic behaviors. Design motifs including highly anisotropic lanthanide ions, low-coordinate transition metals, and radical bridges are used to access unprecedented electronic structures, exceptionally strong magnetic exchange coupling, and anisotropies rivaling those of commercial permanent magnets. They are also creating new systems with potential applications in quantum information science, with the central hypothesis that molecular design principles established for single-molecule magnets can be adapted to give rise to a generation of electronic spin qubits with atomically precise local and global structures.
Porous Polymers and Composite Membranes
They are developing an emerging class of tunable porous network polymers known as porous aromatic frameworks (PAFs) for the selective recovery of diverse solutes for separation and purification applications, such as water remediation and the recovery of high-value metal ions from aqueous waste streams. PAFs exhibit high surface areas and are buttressed by strong covalent bonds, rendering them extremely robust under harsh conditions, including strongly acidic, alkaline, and humid environments. They are also incorporating these functionalized PAFs into polymer membranes to generate high-performance composite membranes for implementation in novel and efficient membrane-based separation strategies.
In the News
Capturing Wellhead Gases for Profit and a Cleaner Environment
National Academy of Sciences Elects Seven From UC Berkeley
A Big Step Toward ‘Green’ Ammonia and a ‘Greener’ Fertilizer
Improved desalination process also removes toxic metals to produce clean water
New technique to capture CO2 could reduce power plant greenhouse gases
A Chain Reaction to Spare the Air
New Material Captures Carbon at Half the Energy Cost
Capturing carbon from power plants is likely in the future to avoid the worst effects of climate change, but current technologies are very expensive. A new material, a diamine-appended metal-organic framework, captures and releases CO2 with much reduced energy costs compared to today’s technologies, potentially lowering the cost of capturing this greenhouse gas.
New material cuts energy costs of separating gas for plastics and fuels
A new type of hybrid material developed at UC Berkeley could help oil and chemical companies save energy and money by eliminating an energy-intensive gas-separation process.
Breakthrough in designing cheaper, more efficient catalysts for fuel cells
UC Berkeley chemists Chris Chang, Jeff Long and Marcin Majda have redesigned catalysts in ways that could have a profound impact on the chemical industry as well as on the growing market for hydrogen fuel cell vehicles.
Berkeley Lab to Develop Novel Materials for Hydrogen Storage
Lawrence Berkeley National Laboratory is aiming to solve how to store enough of hydrogen-powered fuel cells, in a safe and cost-effective manner, to power a vehicle for 300 miles by synthesizing novel materials with high hydrogen adsorption capacities.
Capturing carbon
Researchers at Berkeley and other universities to find ways to capture carbon dioxide, produced by burning coal and natural gas, from the waste stream of power plants so that it can be sequestered underground.
Berkeley Lab to Receive $8.6 Million in Recovery Act Funding for "Transformational" Energy Research Projects
The U.S. Department of Energy (DOE)'s Lawrence Berkeley National Laboratory (Berkeley Lab) has been awarded $8.6 million in Recovery Act funding for what the DOE calls "ambitious research projects that could fundamentally change the way the country uses and produces energy."
$30 million from DOE for carbon capture, sequestration
Two UC Berkeley faculty members will receive $30 million over the next five years from the U.S. Department of Energy to find better ways to separate carbon dioxide from power plant and natural gas well emissions and stick it permanently underground.