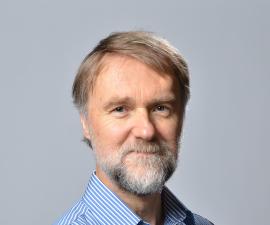
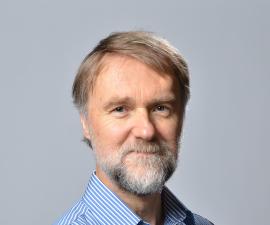
Research Expertise and Interest
physics, standard model of particle physics, symmetries of nature, the symmetry of the electroweak interaction, spacetime symmetries: weak scale supersymmetry, constrained theories for the quark and charged lepton masses, supersymmetric theory
Research Description
What are the fundamental symmetries of nature, and how are they broken?
The standard model of particle physics, while very successful, leaves many fundamental questions unanswered. These questions frequently have to do with symmetries: could certain aspects of particles and their interactions be better understood in terms of a new symmetry? How are these new symmetries, and indeed many of the symmetries of the standard model, to be broken? The symmetry of the electroweak interaction would imply that there is no difference between the left-handed neutrino and the left-handed electron! These particles differ only because the symmetry is broken. The origin of the mass scale of this symmetry breaking is unknown. The difference between the masses of the electron and the muon are a sign that flavor symmetries are broken; but the origin of this breaking is completely unknown. It is remarkable that we can address such problems; in the coming decade we aim to find answers to these fundamental questions of symmetry breaking.
In recent years I have constructed and studied theories with enhanced spacetime symmetries: weak scale supersymmetry, and compact extra spatial dimensions with size from sub-mm to inverse TeV to inverse Planck mass. I have explored the possibility that the breaking of spacetime parity, a remarkable and surprising discovery of the 1950s, is a low energy phenomenon; at larger energies the symmetry is restored, solving the CP problem of the strong interactions. I have worked on grand unified theories in four and higher dimensions, and have considered a variety of frameworks for neutrino masses. I continue to study constrained theories for the quark and charged lepton masses.
The cosmos apparently contains both dark energy and dark matter, contributing in roughly equal amounts to a critical universe. These contents of the universe, as well as the asymmetric baryons and background photons, arise from the underlying theory of particle and gravitational interactions. For all its successes, the standard model of particle physics does not allow the computation of the density of any of these components of the universe. What theory will? I have introduced a wide variety of theories for dark matter, including a general class known as the Freeze-In mechanism.
Current projects:
One aspect of my recent work has been the construction of there's with Higgs Parity that restore spacetime parity symmetry at high energies. These theories predict correlations between the top quark mass and the coupling strength of the strong interaction, motivating precision measurements at colliders. I recently explored the implications of Higgs Parity in the context of grand unification, gravity wave signals, and the electric dipole moment of the neutron. Theories with Higgs Parity also have important implications for neutrino physics, which I am exploring. Higgs Parity theories necessarily contain right-handed neutrinos, and these may produce the cosmological baryon asymmetry via leptogenesis, constraining the scale of parity restoration, and in certain theories possibly explaining why there are three generations of quarks and leptons.
My second recent focus area is axion physics. As has been known for over four decades, these particles can solve the strong CP problem and provide the dark matter of the universe. I have recently discovered alternatives to the standard mechanism for cosmological axion production which have important consequences for the experimental signals. Furthermore, I discovered that a rotating axion field in the early universe can create the cosmological baryon asymmetry. I continue to study these areas.
Why are the lepton flavor mixing angles large while those in the quark sector are small? I am pursuing a variety of ideas to explain this quark-lepton asymmetry. Possible schemes for large mixing between the tau neutrino and the muon neutrino were explored in.
Cosmological data suggest that we live in an interesting period in the history of the universe when the matter, radiation and vacuum contributions to the energy density are broadly comparable. The occurrence of any epoch with such a “triple coincidence” is puzzling, and yet it appears that we happen to live during this special epoch. I have proposed why this might be so, and am following this up with a study of theories which lead to such interesting times.
In the News
Berkeley physics Ph.D. takes “Particle Fever” to the big screen
UC Berkeley alumni, Mark Levinson returned to the Bay Area to premiere his new documentary, ‘Particle Fever,’ about the discovery of the Higgs boson. The film includes Berkeley physicists, Lawrence Hall and Yasunori Nomura, along with several campus alums.
Higgs fever: Overflow crowd hears about new particle
A July 13 lecture and panel discussion drew overflow crowds to hear about the newly discovered Higgs boson. Physicists Beate Heinemann and Lawrence Hall explained the theory and experiment behind this “third” kind of stuff, while three others explored the implications of the discovery.