World’s most sensitive dark matter detector gets even better
The world’s most sensitive experimental search for dark matter has gotten 20 times more sensitive thanks to new techniques that reduce the background interfering with efforts to catch these mysterious particles that comprise 85 percent of all matter in the universe.
The Large Underground Xenon experiment, or LUX, is an 815-pound vat of liquid xenon located nearly a mile underground in the former Homestake gold mine in the Black Hills of South Dakota, near Lead. Detectors surrounding the liquid xenon look for flashes of light emitted when xenon nuclei are hit by weakly interacting massive particles, or WIMPs, which are among the leading candidates for dark matter.
“We look for WIMPs produced in the Big Bang that are still around, up to very high masses — we have the best sensitivity of any experiment to date for WIMP masses above four times that of a proton,” said Daniel McKinsey, one of two spokespeople for LUX, a professor of physics at the University of California, Berkeley, and an affiliate of Lawrence Berkeley National Laboratory. ”We haven’t yet observed dark matter interactions, but the search goes on.”
Dark matter, though invisible to date, is thought to be the dominant form of matter in the universe, whereas all visible matter – stars and gas – comprises a mere 15 percent of the gravitationally bound stuff in the cosmos. Scientists discovered it because of its gravitational influence on the rotation of galaxies and the way it bends light traveling through space.
Scientists’ failure to detect it in Earth-bound experiments, however, suggests that its local interactions are very weak and rare, via the aptly named “weak interaction,” one of the four fundamental forces in the universe. Hence, the name WIMP.
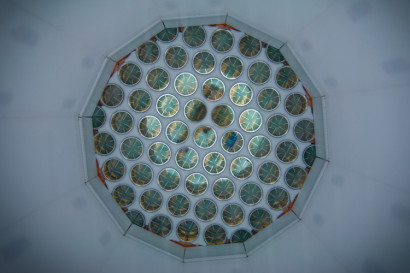
LUX’s new sensitivity allows the scientific team to narrow the range of possible dark matter particle masses and modes of interacting with normal matter, and to eliminate a few hypotheses put forward by theorists as to what this new type of elementary particle truly is.
“It is vital that we continue to push the capabilities of our detector in the search for the elusive dark matter particles,” said Rick Gaitskell, a professor of physics at Brown University and co-spokesperson for the LUX experiment. “We have improved the sensitivity of LUX by more than a factor of 20 for low-mass dark matter particles, significantly enhancing our ability to look for WIMPs.”
The new research is described in a paper submitted to Physical Review Letters and posted to ArXiv. The work re-examines data collected during LUX’s first three-month run in 2013, and helps to rule out the possibility of dark matter detections at low-mass ranges where other experiments had previously reported potential detections.
Looking for dark matter collisions
The LUX collaboration, supported by the U.S. Department of Energy and the National Science Foundation, includes 19 research universities and national laboratories in the United States, United Kingdom and Portugal. It is located in the Sanford Underground Research Facility (SURF) in Lead, a facility owned by the state of South Dakota and supported by the state and philanthropist T. Denny Sanford.
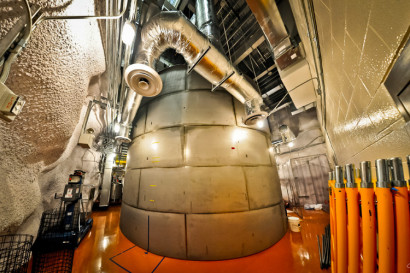
Should a dark matter particle collide with a xenon nucleus, the xenon atom will recoil and emit a tiny flash of light, which is detected by LUX’s light sensors, called photomultipliers. The detector’s location beneath 4,850 feet of rock helps to shield it from cosmic rays and gamma rays that would interfere with a dark matter signal. A jacket of water further shields the experiment, as does the outer two-thirds of the liquid xenon.
The new sensitivity is due to new techniques for calibrating dark matter signals and eliminating bogus collisions by cosmic and gamma rays. One technique involved bouncing neutrons off the xenon atoms to allow scientists to quantify how the LUX detector responds to recoils and better calibrate the response to dark matter, which interacts with xenon nuclei “about a million-million-million-million times more weakly” than do neutrons, Gaitskell said.
They also injected a radioactive gas, tritiated methane, into the detector to generate another type of confounding event, the recoil of an electron, in order to learn how to distinguish these garden-variety events from dark matter.
A second radioactive gas, krypton, was also injected to “better distinguish between signals produced by ambient radioactivity and a potential dark matter signal,” said McKinsey, who is the Georgia Lee Distinguished Professor of Physics at UC Berkeley.
LUX improvements together with advanced computer simulations at Berkeley Lab’s National Energy Research Scientific Computing Center and Brown University’s Center for Computation and Visualization allowed team scientists to test additional particle models of dark matter that now can be excluded from the search.
Future plans
LUX began its current 24/7 run in late 2014 and is expected to continue until June 2016, “representing an increase in exposure of more than four times compared to our previous 2013 run,” McKinsey said. “We will be very excited to see if any dark matter particles have shown themselves in the new data.”
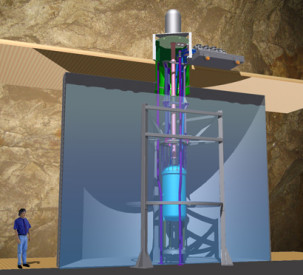
“It’s good to see that the experiments installed in SURF continue to produce world-leading results,” said Kevin Lesko, who oversees SURF operations and leads the Dark Matter Research Group at Berkeley Lab, a member of the LUX collaboration.
Planning for the next-generation dark matter experiment at Sanford Lab is already underway. In late 2016, LUX will be decommissioned to make way for a new, much larger xenon detector known as the LUX-ZEPLIN (LZ) experiment. Compared to LUX’s one-third-ton of liquid xenon, LZ would have a 10-ton liquid xenon target, which will fit inside the same 72,000-gallon tank of pure water used by LUX.
“The innovations of the LUX experiment form the foundation for the LZ experiment, which is planned to achieve over 100 times the sensitivity of LUX,” said LZ spokesperson Harry Nelson of UC Santa Barbara. “The LZ experiment is so sensitive that it should begin to detect a type of neutrino originating in the sun that even Ray Davis’ Nobel Prize winning experiment at the Homestake mine was unable to detect.”
RELATED INFORMATION
- Improved WIMP scattering limits from the LUX experiment (submitted to PRL)
- LUX dark matter experiment
- Brown University press release
- Sanford Lab website
- Daniel McKinsey’s website