Physics Nobel Recognizes Berkeley Experiment on ‘Spooky Action at a Distance’
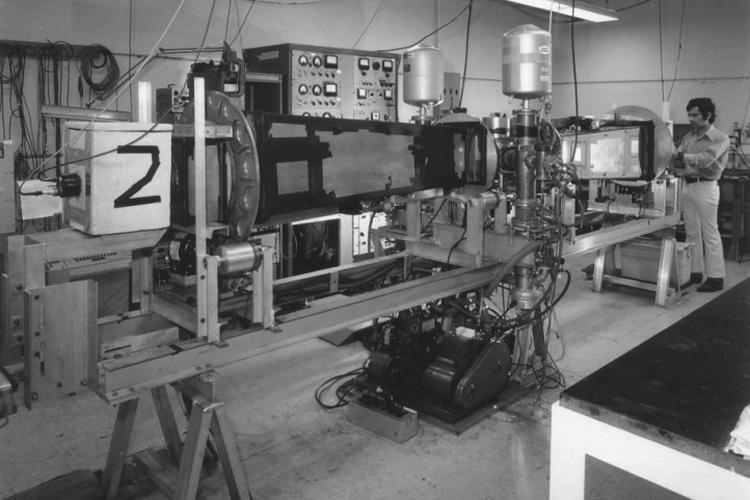
In 1971, graduate student Stuart Freedman and postdoctoral fellow John Clauser took over a room in the sub-basement of Birge Hall at the University of California, Berkeley, and built an experiment that would put to the test one of the most enduring weirdnesses of quantum mechanics, what Einstein called “spooky action at a distance.”
That experiment was the first to show that quantum mechanics really is weird. Two particles, once linked quantum mechanically, or entangled, can be separated by large distances — even the diameter of the universe — and still “know” what happens to one another.
The research was Freedman’s Ph.D. dissertation in 1972, but he subsequently moved onto a broad range of subfields of physics, all related to quantum mechanics, and died tragically in 2012. Clauser continued to refine the experiment to provide more convincing proof that nature violates what’s called Bell’s inequality and prove that the quantum mechanical description of entangled particles is right.
That initial experiment at UC Berkeley and the research it spawned were honored today with a Nobel Prize in Physics to Clauser and two others who followed in his and Freedman’s footsteps: Alain Aspect of the Université Paris-Saclay and École Polytechnique in Palaiseau, France, and Anton Zeilinger of the University of Vienna, Austria. Nobel Prizes are not awarded posthumously, though the Nobel Academy acknowledged Freedman’s role in the research.
“Today we honor three physicists whose pioneering experiments showed us that the strange quantum world of entanglement and Bell pairs is not just the microworld of atoms, and certainly not a virtual world of mysticism or science fiction, but the real world we live in,” said Thors Hans Hansson of the Nobel Committee for Physics during a press conference in Stockholm, Sweden.
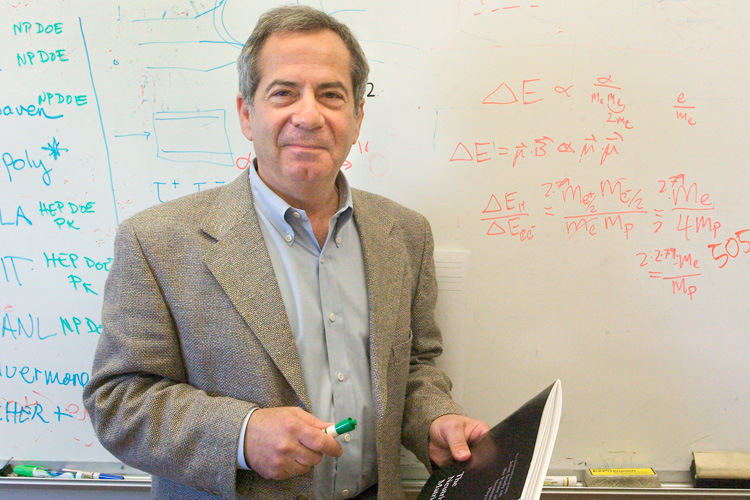
“I am 100% sure Stuart would have received the prize if only he was still with us,” said Freedman’s longtime friend and colleague Dmitry Budker, now a UC Berkeley Professor of the Graduate School in the Department of Physics.
Entangled particles are at the core of today’s quantum computers. Qubits are linked in a single quantum state that, with the right manipulation, could potentially out-compute today’s powerful digital computers. Entanglement at larger distances is also key to China’s quantum-encrypted communications satellite, Micius, which relies on quantum entanglement between photons that are separated by thousands of kilometers.
In the early 20th century, however, quantum mechanics was new, and scientists were confused by the theory’s implications, in particular the idea that the state of a particle — its velocity, location or spin, for example — is unknown until someone measures it. And precise measurement of one aspect of the particle, for example its velocity, inevitably means that another aspect, its location, is less certain. This phenomenon came to be known as the Heisenberg uncertainty principle. While physicists like Neils Bohr argued that quantum mechanics was a complete explanation of nature, many physicists thought quantum mechanics was incomplete and set out to find contradictions.
That group included Albert Einstein, who, in 1935, with two of his postdoctoral fellows, Boris Podolsky and Nathan Rosen, pointed out a seeming paradox in quantum mechanics. According to the theory, two entangled particles remain entangled even when separated from one another. And Heisenberg’s uncertainty principle implies that measuring a property of one entangled particle tells you something about properties of the other entangled particle, no matter how far apart they are. According to Einstein’s theory of relativity, however, light, and thus information, can travel no faster than the speed of light, so simultaneous but separated experiments could not be sharing information. How, then, can a measurement on one affect the state of the other? Is there some hidden variable associated with the particles that allows this to happen?
Subsequently, John Stewart Bell argued that if measurements are performed independently on the two separated particles of an entangled pair, the correlations between the outcomes would differ depending on which view of nature was correct: quantum mechanics, or hidden variable theory.
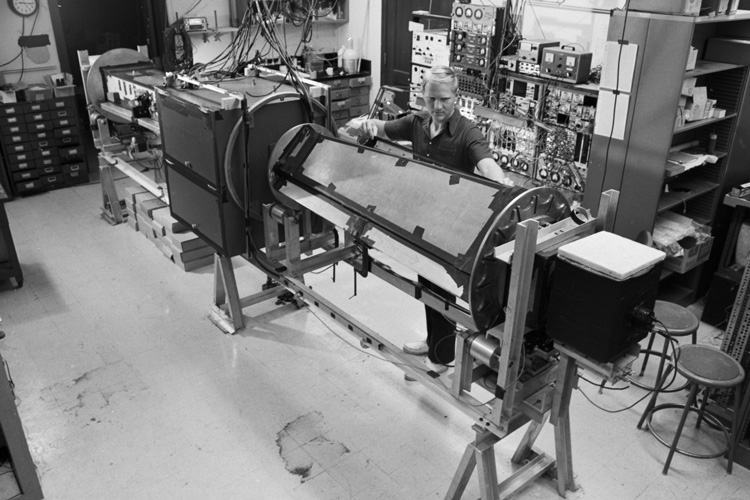
Clauser teamed up with three other physicists — Michael Horne, Abner Shimony and Richard Holt — to propose an experimental test of Bell’s theorem and realized that a technique used earlier at UC Berkeley by the physicist Eugene Commins and his doctoral student, Carl Kocher, might fit the bill. After joining the Berkeley group of Charles Townes as a postdoctoral fellow, Clauser convinced Commins to loan him equipment from the earlier experiment and teamed up with Freedman to scrounge other parts and assemble a new experiment in a basement room.
The experiment involved the decay of excited calcium atoms to produce two photons of light, which, because of the law of conservation of the angular momentum, must have opposite polarizations to make the net angular momentum zero. Since the photons are emitted simultaneously, they are entangled. According to quantum mechanics, measuring the polarization of one should tell you the polarization of the other, even though, in this experiment, the measurements were taken 10 feet apart. If quantum mechanics is incomplete, the correlations between the polarization measurements should be within limits set by what became known as Bell’s inequality.
“I was doing an experiment, essentially by myself, in the basement of Birge Hall,” Freedman recalled in a 2011 talk. “It was a measurement of the polarization correlation from the decay of an atom, and the purpose of doing that was a test of quantum mechanics, it was to see if entangled states behave like quantum mechanics says.”
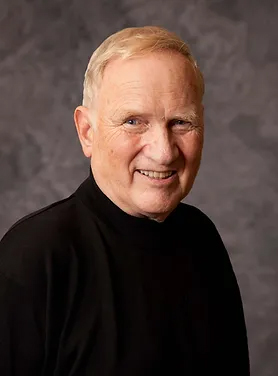
According to background provided by the Nobel Committee, “In 1972, along with doctoral student Stuart Freedman (1944–2012), he (Clauser) was able to show a result that was a clear violation of a Bell inequality and agreed with the predictions of quantum mechanics.”
In the 2011 talk, Freedman showed the data he and Clauser had obtained in 1972 next to that of Aspect’s experiment 10 years later. Both disproved the limits set by Bell’s inequality.
“You can see the data defies that limit, proving that that inequality doesn’t hold in nature and furthermore, it verifies quantum mechanics. This is what we could do in 1972. Ten years later, Alain Aspect, in an experiment in which he used two-photon excitation, was able to do that much better,” Freedman said. “I’ve often wondered about this, whether I should have pursued this, but 10 years convinces me that probably I’d made the right choice.”
Freedman went on to a distinguished career, studying neutrinos and the weak interaction, and eventually returned to UC Berkeley in 1991 to become a professor of physics and a faculty scientist at Lawrence Berkeley National Laboratory. Clauser carried further tests of Bell’s theorem, and is known for the testable version of Bell’s inequality, the so-called Clauser–Horne–Shimony–Holt inequality.
“I went on alone at UC Berkeley to perform three more experimental tests of quantum mechanics,” Clauser said in a recent interview. “All yielded the same conclusions. Bohr was right, and Einstein was wrong.”
Clauser was at UC Berkeley and Lawrence Berkeley National Laboratory from 1969 until 1975, and subsequently worked at Lawrence Livermore National Laboratory and as a consultant and inventor. According to Budker, Clauser returned to Berkeley in the 1990s and, for a few years, worked unofficially in the physics department setting up a novel kind of atom interferometer.
“He always had many interesting and unusual ideas, and was eager to discuss them at great length,” said Budker, who shared the same Ph.D. thesis advisor as Freedman.