Neutron stars may be too weak to power some gamma-ray bursts
A gamma-ray burst is an immensely powerful blast of high-energy light thought to be generated by a collapsing star in a distant galaxy, but what this collapse leaves behind has been a matter of debate.
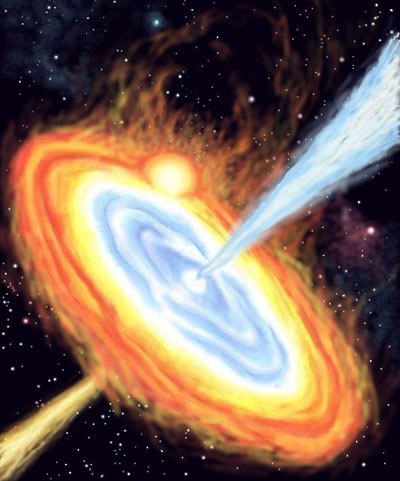
A new analysis of four extremely bright bursts observed by NASA's Fermi satellite suggests that the remnant from a long-duration gamma-ray burst is most likely a black hole – not a rapidly spinning, highly magnetized neutron star, or magnetar since such a burst emits more energy than is theoretically possible from a magnetar.
"Some of the events we have been finding seem to be pushing right up against this total limit for a neutron star progenitor system," said S. Bradley Cenko, a post-doctoral fellow from the University of California, Berkeley.
Cenko will present these findings on Wednesday, Nov. 3, at the Nov. 1-4 Gamma Ray Bursts 2010 conference in Annapolis, Md. Cenko is a member of an international team that includes astronomers from UC Berkeley and the National Radio Astronomy Observatory (NRAO) in New Mexico.
The group has submitted a paper detailing its analysis to The Astrophysical Journal.
Long-duration gamma-ray bursts (GRBs) are presumed to be created by the explosive collapse in distant galaxies of massive stars. The explosion is visible from Earth because the light is emitted in a narrow cone, like a beam from a lighthouse. First discovered in 1967 by satellites looking for nuclear blasts on Earth, gamma-ray bursts have been the focus of several satellite missions, most recently NASA's Fermi gamma-ray space telescope, launched in 2008, and NASA's Swift satellite, launched in 2004.
With accumulating observations, astronomers have been able to create models of how the collapse of a rapidly rotating, massive star can accelerate matter to nearly the speed of light and collimate it into two oppositely directed, tightly focused beams along the spin axis. They have also studied how these particles generate gamma rays and other emissions.
The two leading candidates for powering these long-duration bursts are a magnetar and a black hole, sometimes referred to as a collapsar. In both cases, material from the star falls inward and is catapulted out by the spinning neutron star or black hole. What distinguishes these models is that magnetar-powered bursts cannot be as powerful as black hole-powered bursts.
"The question we have been trying to answer is: What is the true energy release from these events?" Cenko said. "We can measure all the light emitted — very high energy gamma rays, and, at later times, X-ray, optical and radio afterglow emissions — but that doesn't provide a very good estimate, because GRBs emit in relatively narrow jets. We have to get an idea of the geometry of this outflow, that is, how collimated the jets are."
Previous studies have shown that light measured in the afterglow begins to drop steeply at a certain point, and the sooner this drop-off, called a jet break, the narrower the jet. Typically, the gamma-ray burst itself lasts from a few seconds to as long as 100 seconds, but the afterglow, produced when the jets interact with gas and dust surrounding the star, emits visible light for a couple of weeks and radio radiation for several months.
While Swift has observed hundreds of bursts in the past five years and notified astronomers within seconds of detection, the instruments aboard the satellite detect mostly medium-sized bursts that are not as highly collimated and that have a jet break many days or weeks after the burst.
Fermi's Large Area Telescope, however, is sensitive to very bright bursts with jet breaks within several days of the burst, making follow-up observations easier with Swift's X-ray and ultraviolet-optical telescopes and the ground-based Very Large Array, a radio telescope operated by NRAO.
Fermi detects few extremely bright bursts, however — only four in 2009, Cenko said — and does not notify astronomers for nearly a day afterward. Once alerted, however, Cenko's team was able to observe the optical, X-ray and radio afterglow of these four events, find the jet break and use this information, along with the star's distance calculated from its redshift, to estimate the total energy output.
If the energy from these bright bursts were emitted in all directions, it would be equivalent to the mass of the sun being converted instantaneously into pure energy. Because the gamma-ray burst was focused in a cone only a few degrees wide, however, the energies for all four bursts were about 100–1,000 times less than this.
Theoretical models of how these beams are produced place a limit on how much energy a magnetar can generate in one of these explosive bursts: about 100 times less than if one converted the sun entirely into energy. Several of these bright bursts exceed this limit.
“The magnetar model is in serious trouble for such incredibly powerful events,” noted coauthor Alex Filippenko, UC Berkeley professor of astronomy. “Even if the magnetar energy limit is not strictly violated, the tremendous efficiency required by this process strains credulity."
"In the future, we will be trying to make more precise measurements and be looking for more events to rule out a neutron star model," Cenko said.
Cenko and Filippenko’s colleagues are post-doctoral fellows Nat R. Butler and Bethany E. Cobb, astronomy professor Joshua S. Bloom and graduate students Daniel A. Perley and Adam N. Morgan of UC Berkeley; Dale A. Frail of NRAO; Fiona A. Harrison, Mansi M. Kasliwal, Shrinivas R. Kulkarni and Vikram R. Rana of the California Institute of Technology; Joshua B. Haislip, Daniel E. Reichart, Aaron P. LaCluyze and Kevin M. Ivarsen of the University of North Carolina, Chapel Hill; Antonio Cucchiara and Derek B. Fox of Pennsylvania State University in University Park; Edo Berger of the Harvard-Smithsonian Center for Astrophysics in Cambridge, Mass.; Poonam Chandra of the Royal Military College of Canada in Kingston, Ontario; Jason X. Prochaska of the UCO/Lick Observatory at UC Santa Cruz; Karl Glazebrook of the Swinburne University of Technology in Victoria, Australia; Sebastian Lopez of the Universidad de Chile in Santiago; and Max Pettini of the University of Western Australia in Crawley.
The work of Cenko and Filippenko is supported by Gary and Cynthia Bengier, the Richard and Rhoda Goldman Fund, NASA and the National Science Foundation.