Evolutionary arms race
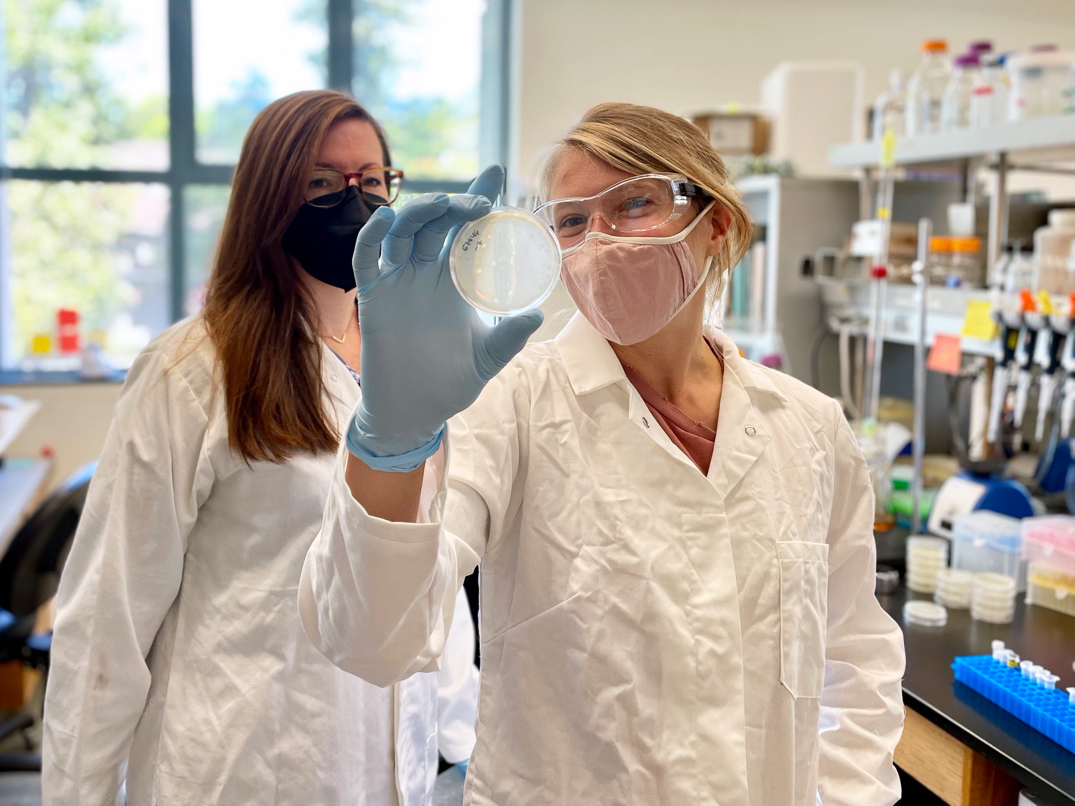
Graduate student Kristen LeGault and assistant professor Kimberley Seed, both in the Department of Plant and Microbial Biology, specialize in the evolution of human pathogens and the viruses that infect bacteria, known as phages.
In partnership with the International Center of Diarrheal Disease Research in Bangladesh, the lab investigates epidemic Vibrio cholerae, a bacterium that causes cholera infections in humans. Cholera evolves defenses against phages, and in turn, phages adapt to overcome bacterial defenses through continued rounds of coevolution. The lab isolates the cholera bacteria and their viruses from patient stool samples from Bangladesh, where cholera outbreaks are common, to better understand the genetic and evolutionary relationship between phages and cholera.
When screening cholera isolates for phage sensitivity, LeGault and Seed found that a strain of cholera had unexpectedly become resistant to infection by the phages. Their analysis led to a key discovery about antibiotic and antiviral defense in cholera, as well as phage adaptation. The findings, appearing in the July 30th issue of Science, outline an important mechanism behind the evolutionary struggle—with implications for treating cholera outbreaks and other bacterial pathogens.
Phage and bacterial evolution
Like in animals and plants, bacterial species like cholera have their own predatory viruses called phages, or bacteriophages. This group of viruses specifically infects bacteria, in the same way that certain viruses specifically target one animal species but not others. By some estimates, the global population of phages outnumbers the bacteria they infect by tenfold.
Bacteria like cholera have developed various sophisticated strategies to neutralize viruses, but phages consistently evolve around these defenses. In an ongoing evolutionary arms race, cholera garners resistance to its phages, and the phages evolve mechanisms that allow it to bypass bacterial resistance and infect cholera.
By isolating the cholera bacteria and phages from patients, and studying how they interact in the lab, researchers can control for confounding factors like temperature, pH, and even other microbes. This base understanding then informs them about how the viruses and bacteria interact in natural environments and humans.
Conventionally, the mere presence of cholera-targeting phages in humans and the environment—for instance, in stagnant or unsanitary water where both bacteria and phage are found, and by which humans are first exposed to cholera infections—has been considered a controlling factor in the spread of cholera outbreaks.
With their new publication, Seed, LeGault, and their co-authors show that whether a phage can control a bacterial population is far more complex—and largely dependent on genetic adaptations in both bacteria and phages.
Understanding phage and antibiotic resistances
To figure out what had changed about cholera to render it resistant to phage infection, the team, helped by former postdoctoral researcher Dr. Steph Hays, compared the genomes of isolates that were sensitive to those that were resistant. To their surprise, the changes in phage sensitivity tracked with changes in the presence and identity of a mobile region of the genome well-known for conferring antibiotic resistance in cholera: SXT ICE. They were excited to discover that different versions of SXT ICEs protect from different phages, suggesting that phages could evolve counter-adaptations to certain SXT ICEs, and may play a role in the fluctuations of SXT ICE abundance seen in their patient samples. Indeed, by looking for phages that can overcome cholera’s defenses, they found a new phage-encoded protein that blocks the action of one of the most common SXT ICE. Through their sampling period, LeGault and Seed found clear examples of coevolution playing out in isolates from human patients.
To address whether SXT ICEs can harbor phage resistances in cholera outside of their sample collection period and region, the researchers got help from staff scientist Angus Angermeyer, who assembled and analyzed a total of 2,600 cholera genomes for the presence of SXT ICEs —the greatest number for any cholera study to date. They found that SXT ICEs, which confer phage resistances, are prevalent in cholera, and new SXT ICEs can emerge and become dominant over relatively short time periods.
SXT ICEs are important to bacterial evolution because they can be quickly transferred to new cells through a process called horizontal gene transfer (HGT). Rather than evolving slowly by mutation and selection, HGT allows bacteria to pick up a whole suite of new functions at once. SXT ICEs are able to move between bacteria, even between distantly related organisms. If a bacterium with an SXT ICE becomes stressed, the SXT ICE takes the stress signal as a cue to get out of a sick host. LeGault, who is the lead author of the study, was curious whether phage infection could act as a stress that would tell the SXT ICE to start mobilizing. This research confirmed that “when a cholera cell gets infected by phages, it stimulates transfer of the SXT ICE, which makes the recipient bacterium resistant to both phages and antibiotics.”
The question of phage therapy and treatment
Scientists used phages to control dangerous and pathogenic bacteria before the existence of antibiotics. With the development of penicillin in 1928—the first mass produced and distributed antibiotic—antibiotics replaced phages due to their effectiveness at controlling bacterial infections.
But in recent decades, due to their overuse, antibiotics have become increasingly ineffective in treating infections. As antibiotic resistance has outpaced antibiotic treatment, researchers are again looking to phages to combat infection. (Some medical centers now rely on phages to treat the most extreme infections, as a last ditch effort where antibiotic resistant infections will almost certainly be fatal. Such treatment is not currently widely used in the medical community.)
These recent findings, says LeGault, caution against the application of phages for treating infections caused by bacteria known to harbor SXT ICEs.
“Now we have a picture that, if you are treating cholera, certain antibiotics and phages will stimulate transfer of SXT ICEs, which can create problems in new cells and even for treating other bacteria,” says LeGault. “One thing you don’t want to do is apply a phage for therapeutics or prophylactics and end up making things worse.”
“Bacteria are going to evolve resistance to phages just in the same way that they evolve resistance to antibiotics, so if we use them therapeutically, bacterial resistance to these phages is potentially a big problem,” says Seed.
Still, by explaining the mechanisms with which phages and bacteria interact, LeGault and Seed’s research could improve treatment using genetically modified phages. For instance, LeGault says that phages could be modified to express inhibitor proteins, allowing the use of phages to control a wider spectrum of cholera strains and other bacterial pathogens.
Seed says that the research highlights the deep need to understand each bacterial pathogen and its phages on a fundamental level, because it can inform how to treat a patient, if and how resistance will develop, and how phages could overcome a bacteria’s resistance barriers. “Our discovery has shown that, as a starting point, a more fundamental understanding of genetics and evolutionary relationships must inform treatment if that treatment is going to be effective,” she says.
RELATED