Berkeley engineers demonstrate unprecedented control of light with a new quantum device
Researchers at the University of California, Berkeley, have developed a new device that allows at-will control over the quantum behavior of single particles of light, or photons, a proof-of-principle demonstration that could form the basis of new optics-based gates for powerful quantum technologies.
The applications possible through the exploitation of quantum computing include analyzing complex molecules that speed drug discovery, processing climate change models for more detailed forecasts and developing sophisticated algorithms for use in encryption and artificial intelligence. The study by Berkeley engineers, recently reported in Nature Photonics and scheduled for the journal’s March 30 print issue, lays a promising path to the development of efficient optics-based quantum gates and circuits, fundamental building blocks needed to power such technologies.
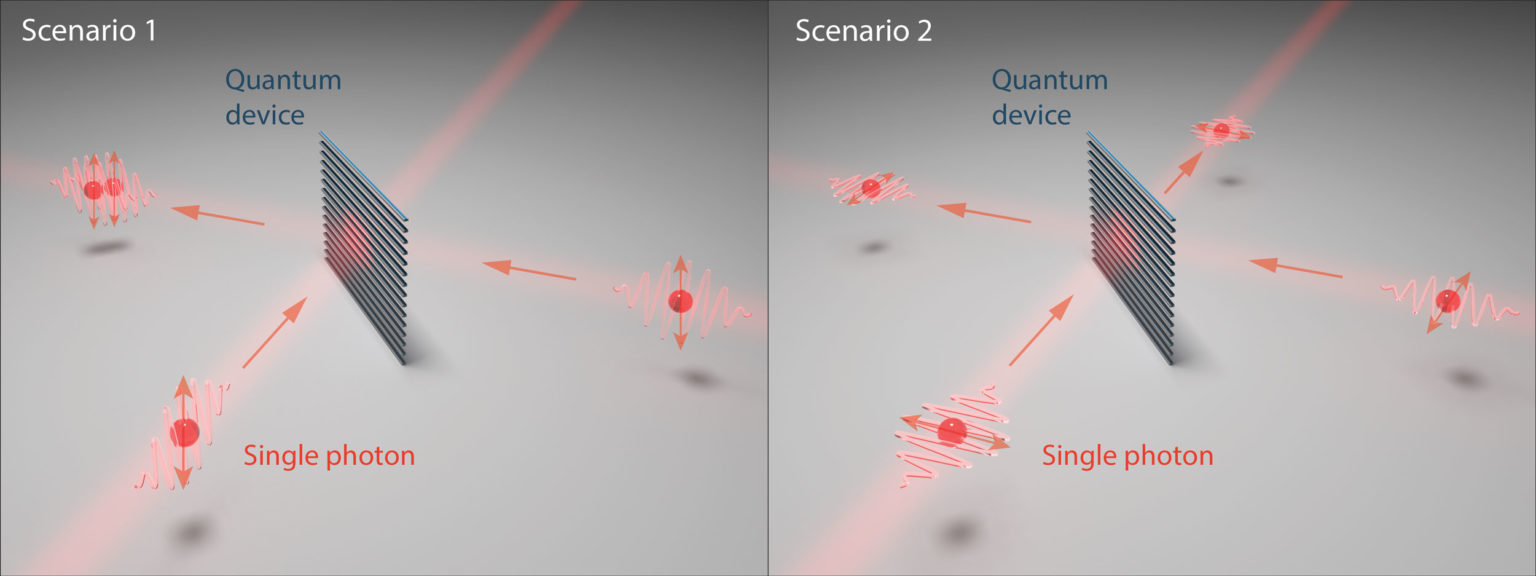
“This is an exciting discovery,” said study principal investigator Xiang Zhang, who is now president of Hong Kong University but completed this research as a mechanical engineering faculty member and director of the NSF Nanoscale Science and Engineering Center at UC Berkeley. “We introduced a new control knob in quantum optics that allows us to manipulate the quantum behavior of single photons. This opens the door to fundamental quantum light-matter interaction and innovative functionalities in quantum technologies.”
For decades, scientists have been developing optics-based systems in classical information technologies because light particles are far better carriers of information than electrons. Light contains more information and can travel farther compared with electricity. A single high bandwidth optical fiber, for example, is equivalent to thousands of electrical wires.
“This is why optical fibers are replacing electrical wires in internet applications,” said study lead author Quanwei Li, who was a Ph.D. student in Zhang’s lab at the time of the study and is now a UC Berkeley postdoctoral scholar.
For quantum applications, harnessing the potential of single photons is one of the major approaches researchers are investigating in the development of quantum computers. It has also become an important barrier to overcome, the study authors said.
Elements of quantum circuits, like their transistor counterparts in classical integrated circuits, must be able to operate as switches for logic functions. In existing optical quantum technologies, beam splitters — half transparent and half reflective optical elements — act as the central element of quantum gates and circuits. As single photons enter, the quantum interference that takes place at the beam splitter always results in the photons emerging together.
That is because in quantum physics, photons are bosons, one of two distinct categories of fundamental particles. Bosons tend to occupy the same space and time, bunching together. In contrast, fermions, the other category of particle — including electrons, protons and neutrons — tend to separate.
Berkeley engineers showed that it is possible to overcome the intrinsic bosonic nature of photons and manipulate how two single photons exit the gate — and to control whether they bunch together or stay apart.
“It has been a major challenge to control the interaction between single photons to build efficient quantum gates — the logic operation unit of quantum computers,” said Li. “Before our study, there had been no easy way to control such quantum interference to achieve desirable switching operations. By introducing a unique optical quantum device, we achieved continuous and dynamical control over the quantum interference of two single photons such that single photons can behave like bosons or fermions or anywhere in between. This kind of versatile switching helps build better photonic quantum gates.”
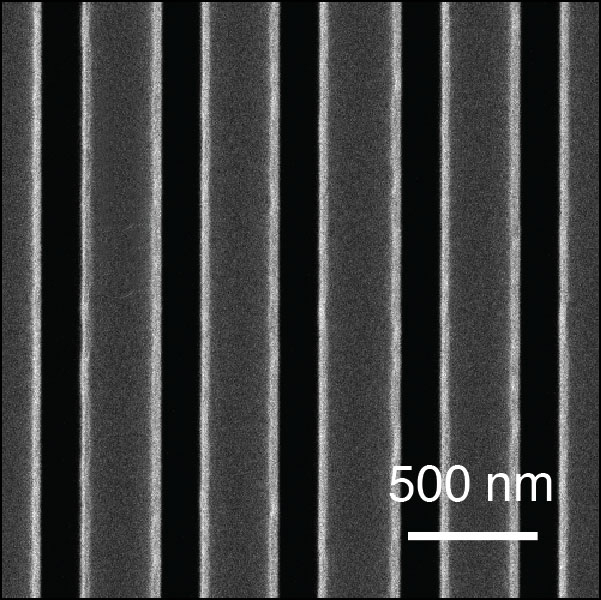
To create the device, the researchers artificially engineered materials with an array of strips smaller than the wavelength of light. Each strip is made up of magnesium fluoride sandwiched between two layers of chromium, measuring 36 micrometers long, 395 nanometers wide and 95 nanometers thick. The thickness is less than one-quarter the wavelength of visible light and one-thousandth the diameter of a human hair.
The researchers found that rotating the strips or the polarization of photons allowed them to control the way single photons exited the device. Instead of bunching together, single photons can remain unattached when leaving the device.
“This metamaterial design is unprecedented, though it might look simple,” said study co-lead author Wei Bao, former postdoctoral researcher in Zhang’s lab and now an assistant professor at the University of Nebraska-Lincoln.
“To the best of our knowledge, there is no existing conventional optical complement or system that can satisfy the same quantum operation we have shown here,” said Li. “Our metamaterial quantum device demonstrates new physics in quantum optics.”
Armed with a new strategy to transform the design of future optical quantum gates, the researchers said the next steps include incorporating the device into large-scale networks to enhance quantum operations.
Other study co-authors at UC Berkeley are Zhaoyu Nie, a Ph.D. student; Yang Xia, a former Ph.D. student; Yahui Xue, a postdoc researcher; and Yuan Wang and Sui Yang, assistant research engineers.
This work is supported by the Gordon and Betty Moore Foundation and the King Abdullah University of Science and Technology.