Berkeley biogeochemist and geomicrobiologist Jillian Banfield studies very, very small things
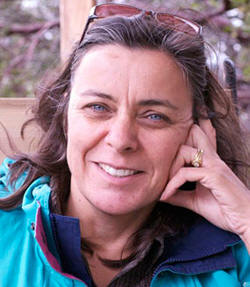
Jillian Banfield studies very, very small things, but her work is vast in its scope and impact. So vast, in fact, that her discoveries have implications for space, the human body and nearly everything in between.
Banfield, a biogeochemist, geomicrobiologist and professor of materials science and engineering, studies microbes—their function and potential both individually and in groups. “If you imagine a cubic centimeter of soil, about the size of the tip of your finger, it could contain a billion microorganisms,” says Banfield, who also holds appointments in the departments of Earth and Planetary Science, Environmental Science, Policy and Management and the geochemistry group at Lawrence Berkeley National Laboratory. “Microorganisms are essentially everywhere, and they carry out all the really essential transformations that drive earth’s biogeochemical cycles.”
Microscopic organisms are so diverse and abundant that entire new genetic branches are still being discovered. What do these tiny microbes do? What roles do they play in their communities? Finding answers to these questions and developing methods of using microbes to solve urgent environmental and medical problems are at the heart of Banfield’s research.
Last fall, Banfield received two of the most prestigious awards in her field—the Benjamin Franklin Medal in Earth and Environmental Science and L’Oreal-UNESCO “For Women in Science” award—for groundbreaking work on how microbes alter rocks and interact with the natural world.
In 2004, she helped pioneer a research tool called “shotgun metagenomics,” which reconstructs genomes from microbial DNA extracted directly from environmental samples. The bacterial DNA sequence they were able to create, from an acid mine drainage system, resulted in nearly complete genomes for bacteria that had previously resisted being cultured in the lab.
From that point, it became possible to study entire genomes without the barriers and obstacles of working with laboratory cultures. There are other research benefits as well. “One of the real powers of metagenomics is that we don’t have to know what we’re looking for,” she says. “There’s so much out there that we don’t know. Some microbes make enzymes or molecules that we didn’t even know about before, using pathways we didn’t know existed. All of this is useful for understanding life activity on earth.”
A native of Australia, Banfield earned her bachelor and master’s degrees from Australian National University, and afterward worked as a geologist for an Australian mining company. Later, she came to the United States to pursue a doctorate in earth and planetary sciences from Johns Hopkins University. She worked at the University of Tokyo and the University of Wisconsin–Madison before joining the Berkeley faculty in 2001 and engineering in 2005. Her work floats at the interfaces of many scientific disciplines, using the methods and approaches of biology, physics and geochemistry.
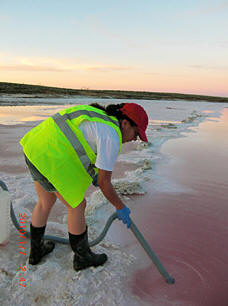
The metagenomic tool has broad applications, working equally well on samples collected from hostile terrestrial environments as well as those taken from deep within the human body. One ongoing project looks at microbes in the guts of premature human infants.
Vulnerable to often-fatal infection, preemie intestines also offer a rare sterile environment upon birth. Banfield and colleagues at the University of Pittsburgh and Stanford University sequenced and reconstructed the genomes of most intestinal microbes from a human infant gut and then documented how the microbe populations changed over time. Further studies could help scientists understand illnesses like necrotizing enterocolitis—a condition that tends to affect newborns and leads to the death of intestinal tissue.
“We are also looking to find out where the organisms that colonize the premature infant gut come from, especially those with the potential to make the babies sick,” Banfield says. “Did they come from air in the hospital room, the gloves, the incubator?”
Banfield is passionate about the adaptability of her research. To study human infants, she and her team used the same approach they employed studying a polluted aquifer in Rifle, Colorado.
Rifle’s groundwater is contaminated as a result of years of uranium and vanadium ore-processing. Banfield and colleagues are contributing to a large multi-disciplinary project at the site, selectively introducing microbes to the aquifer as a way to break down pollutants. Organic carbon, for example, promotes the growth of bacteria that reduce iron and uranium. By setting this process in motion, researchers can investigate ways to clean the toxic groundwater and prevent uranium from continuing to disperse.
“We can make microbes do what we want if we understand how they work,“ Banfield says. “We are figuring out what to add and in what quantities. In an intelligent way, like moving the puppet strings on the surface, we can stimulate the subsurface and carry out a transformation.”
Her research group is also studying microbial communities in Lake Tyrrell in southeastern Australia. A vivid pink, hyper-saline lake that produces a salt crust in summer, Lake Tyrrell provides an opportunity for scientists to understand how groups of microorganisms behave under harsh conditions. Working as part of an international collaborative group, the researchers hope to learn how microbial communities cope with the simultaneous challenges of high heat and salt concentrations.
It’s yet another example of how Banfield’s research into the tiniest of organisms might one day yield substantial advances in understanding our world and bettering our lives.