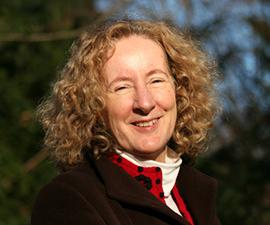
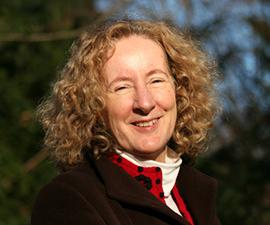
Research Expertise and Interest
quantum information and quantum computation, control and simulation of complex quantum systems, quantum effects in biological systems, quantum physics, quantum mechanics
Research Description
Professor Whaley's research lies at the interface of quantum physics with information and computation, with chemistry, and with biology. Her work focuses on quantum information and quantum computation, control and simulation of complex quantum systems, and quantum effects in biological systems. Quantum information processing employs superposition, entanglement, and probabilistic measurement to encode and manipulate information in very different ways from the classical information processing underlying current electronic technology. Theoretical research in Professor Whaley's group in this area is focused in quantum algorithms, quantum control, quantum information and quantum measurement, analysis and simulation of open quantum systems, macroscopic quantum states and quantum metrology. Specific topics of current interest include quantum feedback control, algorithms for near term quantum computation, and analysis of macroscopic quantum superpositions in interacting many-body systems. Such superposition states, dramatically illustrated by Schrodinger's famous cat paradox, offer unprecedented opportunities for precision measurements. Professor Whaley's research in quantum biology seeks to characterize and understand the role of quantum dynamical effects in biological systems, with a perspective that combines physical intuition and detailed quantum simulation with insights from various branches of quantum science – quantum physics, molecular quantum mechanics and quantum information.
In the News
UC Berkeley to lead $25 million quantum computing center
Quantum expert Birgitta Whaley appointed to White House science advisory council
Scientists Set Quantum Speed Limit
The flip side of Heisenberg’s uncertainty principle, the energy time uncertainty principle, establishes a speed limit for transitions between two states. UC Berkeley physical chemists have now proved this principle for transitions between states that are not entirely distinct, allowing the calculation of speed limits for processes such as quantum computing and tunneling.