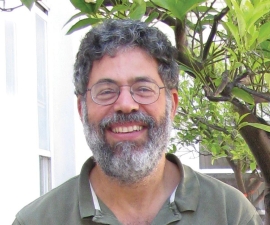
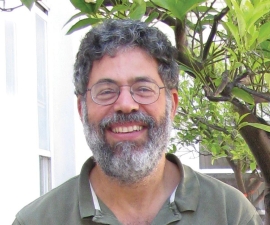
Research Expertise and Interest
astrophysics, plasma processing, physics, basic plasma physics, non-neutral plasmas, basic plasma physics experiments, pure electron plasma traps, cyrogenic plasmas, plasma bifurcations, basic non-linear dynamics, autoresonance
Research Description
Joel Fajans is a professor in the Department of Physics.
Although most of the universe is in the plasma state, the basic properties of plasmas are not well understood. Plasmas are collective systems, and exhibit remarkably complicated nonlinear behaviors like turbulence and chaos. Better understanding of basic plasma physics should help us in areas as diverse as astrophysics and plasma processing.
His research group studies an unusual type of plasma—non-neutral plasmas. Such plasmas consist of particles of only one sign of charge: typically electrons, ions, positrons, or antiprotons. Since non-neutral plasmas are extraordinarily stable, readily controllable, and easily diagnosed, they are ideal for many basic plasma physics experiments. In addition, non-neutral plasmas can be used for experiments on fluid dynamics nonlinear dynamics and antihydrogen formation. As non-neutral plasmas are confined in small scale traps, experiments are run by one or two people.
Current projects
Currently our research is focused on three problems:
Antihydrogen formation: One of the most exciting recent physics accomplishments was the production of antihydrogen at CERN. The antihydrogen was synthesized from positron and antiproton plasmas quite similar to the electron plasmas that we make in our lab. Ultimately, researchers intend to look for CPT violations by comparing the spectra of hydrogen and antihydrogen, and, possibly, anomalous gravitational effects on antimatter. However the researchers at CERN were only able to create antihydrogen, not trap it, and the most interesting physics can only be done on trapped antihydrogen. We have joined a new group, ALPHA http://alpha.web.cern.ch/alpha/ whose goal is to trap antihydrogen, and are currently running tests at Berkeley on trapping schemes.
BGK waves: Recently we discovered a method of generating very large, very high Q structures in our plasmas. These structures are related to BGK waves. BGK wave theory underpins much of theory of nonlinear waves in plasmas, but have rarely been observed, and never with such high Q or high amplitude. They are related to the structures seen in space plasmas and in laser-plasma interactions. We have been studying the details of these waves, particularly their harmonic content, lifetime, and excitation method.
Autoresonance: Autoresonance is a powerful, very general technique for exciting nonlinear oscillators. We study this technique in experiments using our plasmas, analytic theory, and numerical simulations.
In the News
Antimatter Embraces Earth, Falling Downward Like Normal Matter
Is antimatter anti-gravity?
Antimatter is strange stuff. It has the opposite electrical charge to matter and, when it meets its matter counterpart, the two annihilate in a flash of light.
The First Spectroscopic Measurement of an Anti-Atom
Scientists at Lawrence Berkeley National Laboratory have played leading roles in designing and operating ALPHA, the CERN experiment that was the first to capture and hold atoms of antihydrogen, a single antiproton orbited by a single positron.
CERN group traps antihydrogen for more than 16 minutes
The ALPHA experiment at CERN in Geneva has successfully trapped rare antihydrogen atoms for 1,000 seconds, or more than 16 minutes. This is long enough to start experimenting for the first time on antimatter atoms to determine whether they act like normal matter.
Antihydrogen trapped for first time
The particle accelerators at CERN in Geneva produce scads of antiprotons, which five years ago were combined at high speed with positrons to create for the first time antimatter atoms: antihydrogen. Those atoms annihilated with normal matter within microseconds, but an international team involving UC Berkeley and LBNL physicists has succeeded in slowing such atoms down and trapping them for a tenth of a second. This will allow experiments on a type of matter that hasn't been available since shortly after the Big Bang 14 billion years ago.