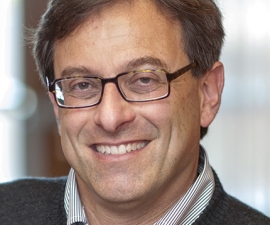
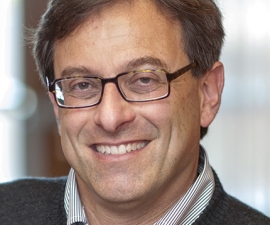
Research Expertise and Interest
Ion channel function, synaptic plasticity, neural excitability, synaptic transmission, the synapse
Research Description
Ehud Isacoff is a professor of neurobiology in the Department of Molecular & Cell Biology. The work in his lab is focused on in four intersecting areas: a) Mechanisms of ion channel function, b) Synapse development and plasticity, c) Neural circuit function, and d) Design of novel probes for the optical detection and manipulation of neuronal signaling. The projects all involve optical spectroscopy, imaging and electrophysiology.
In the News
2025 MTI Innovator Awardees Announced
Weill Neurohub will fuel race to find new treatments for brain disease
With single gene insertion, blind mice regain sight
National Academy of Sciences Adds Five Berkeley Faculty Members to its Ranks
Editing brain activity with holography
$21.6 million funding from DARPA to build window into the brain
New therapy holds promise for restoring vision
NIH awards UC Berkeley $7.2 million to advance brain initiative
The National Institutes of Health today announced its first research grants through President Barack Obama’s BRAIN Initiative, including three awards to the University of California, Berkeley, totaling nearly $7.2 million over three years.
Three Bay Area institutions join forces to seed transformative brain research
Two state-of-the-art research areas – nanotech and optogenetics – were the dominant theme last Thursday, Sept. 18, as six researchers from UC Berkeley, UC San Francisco and Lawrence Berkeley National Laboratory sketched out their teams’ bold plans to jump-start new brain research.
On Memory’s Trail
Ehud Isacoff and his colleagues explore the brain at several levels critical to ultimately understand how memories form and what can threaten their demise. He is the Director of Berkeley’s Helen Wills Neuroscience Institute.
Neural circuit ensures zebrafish will not bite off more than it can chew
UC Berkeley neuroscientists have found that when zebrafish larvae see large objects, like leaves or other zebrafish, inhibitory nerve cells fire in the brain to tamp down a prey response. But when the larvae see small, prey-size objects, fewer inhibitory nerve cells fire and the fish quickly responds. This simple neural circuit helps explain the visual filters that enable prey capture.