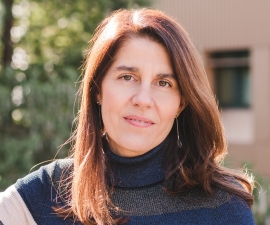
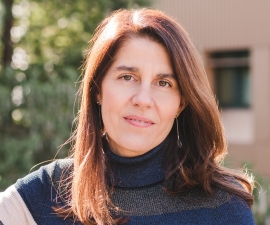
Research Expertise and Interest
materials science, ultrafast science, quantum materials, sustainable materials, topological phases of matter
Research Description
Prof. Lanzara interests are in exploring the science and technology of sustainable materials; quantum materials such as superconductors, two dimensional and topological heterostructures; and novel biological processes. Through development and application of advanced scientific tools, coherent optical laser drives and artificial intelligence, her laboratory is at the forefront of materials science and condensed matter physics. She is also applying these new advanced tools to explore fundamental processes in biology.
Lanzara is the founding director of the Berkeley Center for Sustainable Materials and Innovation. She also directs Berkeley Discovery, a campus wide initiative to launch undergraduate research ventures.
Other affiliation of Prof. Lanzara include the Kavli Energy Nanoscience Institute, the department of Applied Sciences and Technology, the Bakar Institute of Digital Materials for the Planet and the Materials Sciences Division at LBNL.
In the News
Study Shows How Light Can Transform an Insulating Material Into a Semimetal
Scientists Create a Longer-Lasting Exciton that May Open New Possibilities in Quantum Information Science
From EarEEG to Quantum Computing, Bakar Prize Winners Go for Broke
Nine Faculty Members Elected to American Academy of Arts & Sciences
Spin-TOF: A One-of-a-Kind Tool for Studying Spin-Dependent Electronic Properties
Searching for quantum weirdness in interactions between light and matter
Seven new Bakar Fellows already are making an impact
Revealing Hidden Spin: Unlocking New Paths Toward High-Temperature Superconductors
A Quick Look at Electron-Boson Coupling
Spin-Resolved ARPES Envisaged for the Advanced Light Source
A new tool to attack the mysteries of high-temperature superconductivity
Using ultrafast lasers, Berkeley Lab scientists have tackled the long-standing mystery of how Cooper pairs form in high-temperature superconductors. With pump and probe pulses spaced just trillionths of a second apart, the researchers used photoemission spectroscopy to map rapid changes in electronic states across the superconducting transition.
Featured in the Media
New scientific and technological innovation hub established at UC Berkeley by the Enel Foundation and the Center for Sustainable Materials and Innovation (CSMI), will “solve some of the most pressing sustainable development goals around materials design and the circular economy”, according to Alessandra Lanzara, head of CSMI.