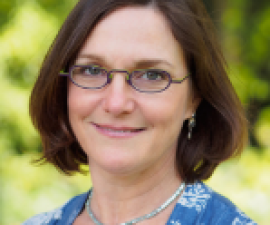
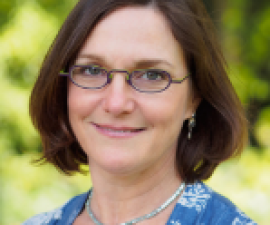
Research Expertise and Interest
chromosome structure and function, higher order chromosome structure, cell cycle controls, chromosome remodeling and reorganization during meiosis, spatial pattern formation in eukaryotic and prokaryotic cells, genome mapping, eukaryotic genomes, whole-genome analysis
Research Description
A major event in the evolution of life on earth was the appearance of meiosis, a special cell division process that enables sexual reproduction. During meiosis, the two copies of each chromosome inherited from the two parents physically interact through a highly choreographed process of pairing, synapsis, and recombination. This results in the mixing of genetic traits, and is essential for an organism to make reproductive cells such as sperm, eggs, and pollen. Errors in this process are a major cause of human birth defects such as Down Syndrome. The Dernburg Lab uses genetics, microscopy, genome editing, and genome-wide mapping to understand the mechanisms that ensure faithful chromosome segregation during meiosis. They also seek to understand how meiosis contributes to genome stability and evolution.
Current Projects
Quality control during oogenesis. Proper execution of meiosis is essential for fertility and prevention of chromosomal birth defects. Work in our lab and others has uncovered a number of meiotic "checkpoints," surveillance mechanisms that monitor cellular events and delay the cell cycle or lead to programmed cell death when errors arise. In 2005 we reported the discovery of a "synapsis checkpoint" that leads to the death of oocytes if chromosomes fail to undergo synapsis. We later noted that failures in synapsis result in persistent localization of a meiotic kinase, PLK-2, at chromosome pairing centers, as well as a prolonged interaction between these chromosome regions and the nuclear envelope. We wondered if this might be a molecular trigger for apoptosis. Using a novel auxin-induced proximity system, we have found that indeed, the presence of PLK-2 at chromosome pairing centers or the nuclear envelope is sufficient to trigger apoptosis of oocytes. We have further found that phosphorylation of the nuclear lamina results in "softening" of the nuclear envelope, and that the resulting distortions of the nuclear surface are detected by mechanosensitive Piezo ion channels, probably residing within the nuclear membrane. We suspect that a similar pathway helps to ensure proper chromosome segregation in human meiosis. (Liu et al., manuscript in preparation).
Crossover regulation. A longstanding mystery about meiosis is how cells ensure that each chromosome pair undergoes "crossing-over," a recombination process that creates physical links between chromosome pairs and is essential for faithful segregation. Each chromosome pair normally has at least one crossover, yet there are very few "extra" crossovers - i.e., crossovers show a highly nonrandom pattern across the genome. In 2017 we reported a surprising discovery: the synaptonemal complex, a specialized protein polymer that assembles between chromosomes during meiosis, is an unusual liquid crystalline material. More recently, we found that some crossover proteins can diffuse along this unusual interface between chromosomes. These crossover factors appear to undergo condensation and coarsening through diffusion along the synaptonemal complex. This led us to propose a model that can explain the genome-wide patterning of crossovers observed in most eukaryotes.
Untangling the role of DNA topology in meiosis and genome evolution. Sexual reproduction is a complex and costly process. It has never been clear how the benefits of meiotic recombination compensate for the "2-fold cost" of sex. We are currently testing a new idea: that meiosis may promote genome stability by editing regions that are prone to DNA damage due to topological conflicts. To probe this idea, we are developing new methods to map DNA supercoiling across the genome and to study the roles of DNA topology during meiosis. Support for this idea could explain how meiosis led to the evolution of large, complex genes and genomes in eukaryotes.