Researchers Awarded $3.7M To Build Next-Generation Haloscope in Search for Dark Matter
Funding will support “tabletop” physics that could change our understanding of the universe
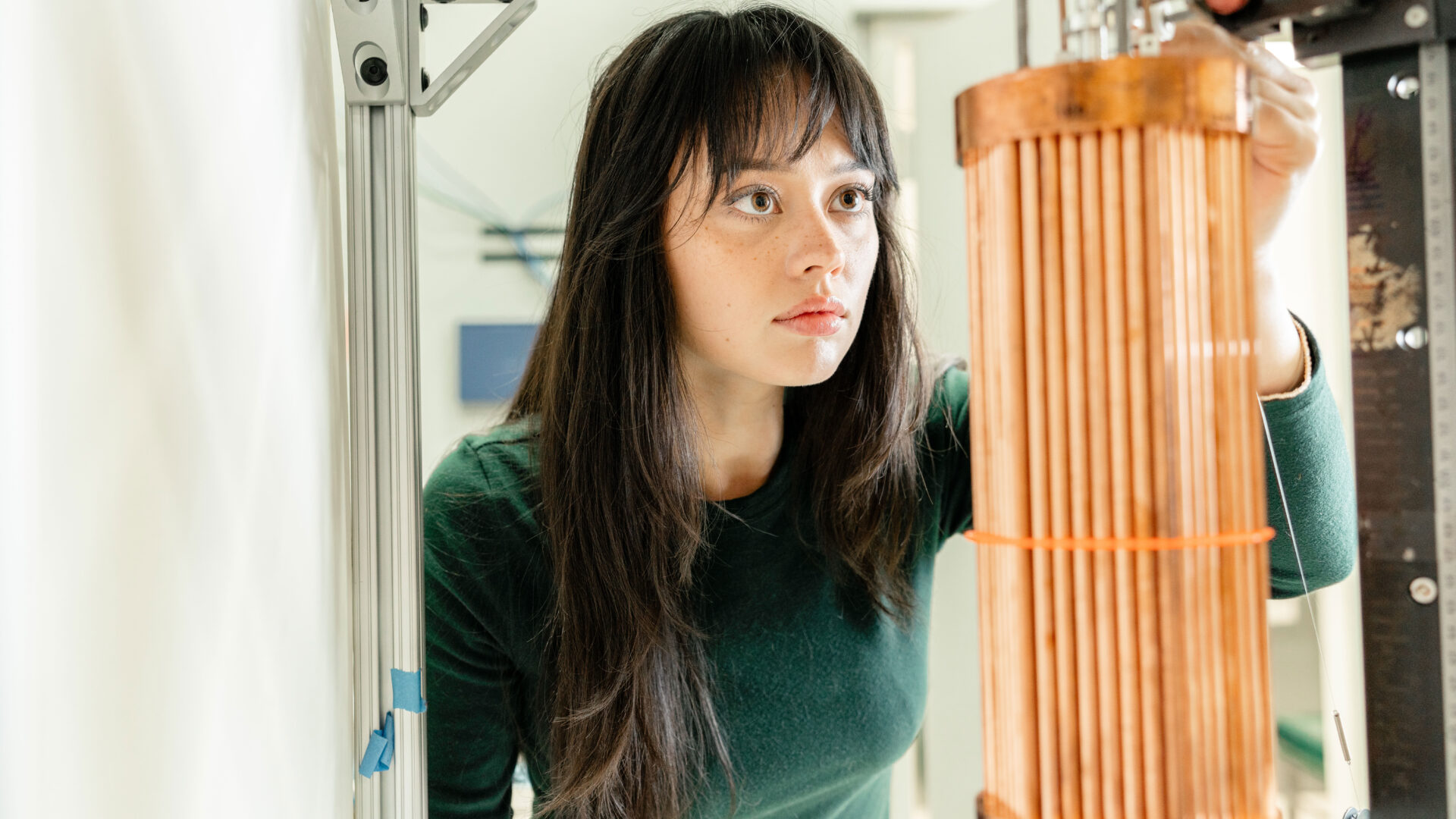
A team of researchers, hailing from nearly a dozen institutions and led by UC Berkeley professor of nuclear engineering Karl van Bibber, has been awarded $3.7M to continue their quest for the elusive axion, a particle believed to make up dark matter in the universe.
This project is among 11 “tabletop” experiments funded through a collective five-year, $30M pledge by the Gordon and Betty Moore Foundation, the Simons Foundation, the Alfred P. Sloan Foundation and the John Templeton Foundation. Small in size but big on science, these innovative experiments “aim at expanding the frontiers of fundamental physics while still fitting into a typical room-sized university physics research lab.”
The newly funded project, “A Plasma Haloscope for the Post-Inflation Axion,” will bring together researchers from UC Berkeley, Yale, University of Colorado Boulder, MIT, Johns Hopkins, Wellesley, Arizona State University, Stockholm University, ITMO University, Cambridge and Oak Ridge National Laboratory. It was selected from hundreds of proposals and seeks to build a new dark matter experiment, called ALPHA, in an existing 16 Tesla magnet at Yale.
“We’ll be looking for the dark matter in the universe, in particular one candidate — an ultra-light, hypothetical elementary particle called the axion,” said van Bibber. “But we’ll be looking in an entirely new, much higher mass range than has been searched for previously.”
This grant will enable van Bibber’s team to advance dark matter research by pushing the boundaries of quantum detection and microwave technology. Leveraging their collective expertise across multiple domains, the researchers will build a next-generation haloscope outfitted with a powerful superconducting magnet, a novel metamaterial resonator, and a quantum sensing technique capable of detecting signals as low as a trillionth of a trillionth of a watt, known as squeezed-vacuum states.
Four decades ago, researchers realized that exposing axions to a strong magnetic field would cause them to decay into single photons with an extremely tiny probability, but possibly just large enough to enable their detection. This conversion process can be boosted by a microwave cavity, which acts like an electromagnetic version of a bell or tuning fork. The new metamaterial resonator, consisting of a 2D array of very thin wires, will instead provide this resonance at the researchers’ target frequencies of 10 to 20 GHz, where they will initially scan for dark matter, and later, absent discovery, up to 50 to 100 GHz.
Dark matter consists of particles that do not interact, absorb, emit or reflect light, making it impossible to see, except to observe its effects on other objects in the universe. The mystery of dark matter dates back to 1933, when Caltech’s Fritz Zwicky first noticed the unusually rapid motion of galaxies within rich clusters of galaxies, presumed to be gravitationally bound. Detailed measurements of the rotation speeds of spiral galaxies — such as our own Milky Way, by Vera Rubin and Kent Ford in the 1960s — finally put the existence of dark matter on a firm footing. Today, researchers believe that the universe is about 95% dark, consisting of 68% dark energy and 27% dark matter, with ordinary matter making up a mere 5%.
Axions, the subatomic particles believed to make up dark matter, are extremely light, with about a trillionth of an electron’s mass, and very dense, estimated to be 100 trillion per cubic centimeter locally. But the axion is also extraordinarily weakly interacting, second only to gravity. Ironically, the prediction of the axion sprang from a theory to remedy a loose end in the theory of the strongest force in the Standard Model of particle physics, namely the unexpected absence of an electric dipole moment of the neutron.
Hunting for these particles has proved challenging for scientists, but van Bibber remains upbeat. “If we find an axion as a result of this experiment, we’ll actually solve two problems in fundamental physics,” he said. “One is the strong charge-parity symmetry problem, and the other is the mystery about what dark matter is. I’ve been described as clinically optimistic, which I suppose is a virtue in tackling a century-old problem.”