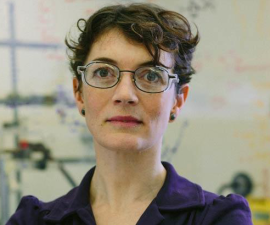
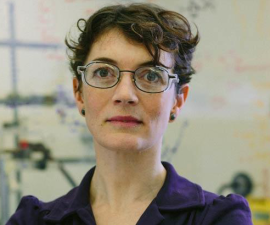
Research Expertise and Interest
synthetic chemistry, inorganic chemistry, organometallic chemistry, actinide, rare earth catalysis
Research Description
Polly Arnold is a professor in the Department of Chemistry. Her research group is focused on reactive and unusual complexes of the rare earths and actinides. The group targets new rare earth based molecular catalysts for difficult transformations such as hydrocarbon C-H bond functionalisation and conversions of abundant small molecules including dinitrogen. The electronic structure and bonding information gained from these studies also contributes to a better understanding of these poorly-understood but relatively earth-abundant heavy elements. This is important for the sustainable separation and recycling of technology critical rare earths that are needed for energy-relevant technologies, and for the safe stewardship of our nuclear waste legacies. The Arnold group has state-of-the-art facilities and capabilities for the synthesis and study of highly air-sensitive complexes, and the isolation of molecules with previously unseen electronic structures and reactivities from across the f-block.
Homogeneous rare earth catalysis
Understanding, controlling, and predicting the subtle interactions that traditionally inert molecules such as dinitrogen, carbon dioxide and hydrocarbons form with metal cations is a major challenge in molecular science, and a key technology enabler in areas such as homogeneous catalysis, drug recognition, polymer properties, and metal recovery. The 4f-row, the rare earths, whose salts are as common as iodine and ten times less toxic than those of iron, offer great potential for the catalytic conversions of simple inert molecules needed for a sustainable, renewable-based future. The group has shown many new small molecule reactivities previously considered impossible for the f-block, and not observed in the d-block.
The group are studying the first f-block catalysts for the catalytic conversion of atmospheric dinitrogen, N2, to amines. They have also investigated reversible CO2 insertion and the subsequent synthesis of cyclic carbonates from CO2 and epoxides at r.t.p. as well as exceptionally fast and controlled ring opening polymerization of cyclic lactones to biodegradable polyesters. Bimetallic systems with tunable Ln-Ln distances are being used to test hypotheses in copolymerisation reactions.
Actinide and transuranic synthesis and reactivity
Fundamental studies on f-block complexes with unusual electronic structures can help explain exciting phenomena at the quantum level such as single molecule magnetism, single-molecule Kondo behaviour, and unconventional superconductivity, potentially providing transformative breakthroughs for quantum computing and future technologies at the nano-scale. The challenges of paramagnetism, radiotoxicity, and relativistic effects have hampered the use of traditional investigative methods or calculations alone to quantify and exploit the structural and electronic subtleties that govern these behaviors. For example, molecular f-block N2 complexes were considered unisolable until recently, even though Haber noted that uranium was better than Fe as a heterogeneous catalyst for the industrial fixation of atmospheric dinitrogen to ammonia, the Haber-Bosch process. The group has now made over half the known actinide dinitrogen complexes and used these simple systems to understand the complex orbital contributions that stabilize the binding.
Most recently, studies on new transuranic organometallic complexes are using new techniques to explore and even manipulate the different orbital contributions to covalency.
Uranyl oxo chemistry and catalysis
The oxo groups of the d0f0 U(VI) uranyl dication were generally considered inert and to have no relevant chemistry until a decade ago when the group used Lewis acid- binding to activate the uranyl unit, reporting the first covalent O-element bond formation at a uranyl ion. Since then the group has learnt how to control 1-and 2-electron reduction and/or uranyl oxo- functionalization with elements from across the whole periodic table, from hydrogen to plutonium (including the first transuranic-uranyl complex), defining the mechanisms and rules that control uranyl oxo-elementation and metalation. The work has opened up routes to more environmentally relevant systems, and more new studies of transuranic analogues.
Most recently, the group has also developed the controlled catalytic photochemical C-H bond functionalisation by well-defined uranyl complexes.