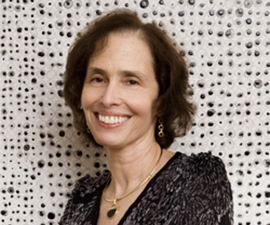
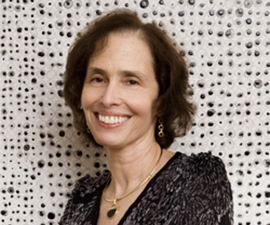
Research Bio
Barbara Meyer is a professor in the Department of Molecular and Cell Biology. Research in the Meyer Lab addresses basic aspects of chromosome dynamics and developmental biology: how chromosomes fold and align to achieve proper segregation; how gene expression is coordinately regulated across the entire X chromosome; how choices are made between alternative cell fates; how regulatory gene hierarchies specify sexual fate. Their studies of X-chromosome-wide gene expression through the process of dosage compensation have revealed a mechanistic link between higher-order chromatin structure and chromosome segregation during meiosis and mitosis. These studies have led to an analysis of chromosome structure and crossover control during meiosis and macromolecular structures called kinetochores that attach and move chromosomes within the spindle during chromosome segregation.
Current Projects
Counting X chromosomes and autosomes to determine sex. In numerous organisms, the decision to become male or female is made by an X-chromosome counting mechanism that reliably distinguishes one X chromosome from two. We have used the model organism Caenorhabditis elegans to dissect such a precise chromosome counting mechanism in molecular detail. In this nematode, the two-fold difference in X-chromosome dose between males (XO) and hermaphrodites (XX) is translated into the non-linear response of the target gene xol-1 (XO lethal), a switch that specifies male fate when active and hermaphrodite fate when inactive. Setting xol-1 expression is essential for viability as well as sex determination because xol-1 also controls X-chromosome dosage compensation. Five X-linked genes called X-signal elements communicate X-chromosome dose by controlling the activity of xol-1, using a combination of at least two mechanisms. One involves transcriptional repression of xol-1 in XX embryos and requires an X-signal element that encodes a nuclear hormone receptor. A second involves the post-transcriptional regulation of xol-1 and requires an X-signal element that encodes an RNA binding protein. This protein represses xol-1 by preventing the RNA processing event that generates the functional xol-1 transcript. Both the transcriptional and RNA processing mechanisms of repression act together to ensure the fidelity of the X-chromosome counting process. Sex is not determined simply by X-chromosome number, but rather by the ratio of X chromosomes to sets of autosomes. We have shown that the autosomal signal is composed of autosomal signal element genes, one of which encodes a T-box class of transcription factors and the other encodes a protein with glutamine and asparagine-rich repeats, zinc fingers, and a metalloprotease domain. How these autosomal signals are integrated and functionally oppose the X signals to regulate sexual fate is a focus of future studies. Efforts are also directed toward defining other X and autosomal signal element genes.
Dosage Compensation and Chromosome Segregation. In flies, worms and mammals, specialized dosage compensation complexes are targeted exclusively to the X chromosomes of one sex to modulate transcript levels by altering chromosome architecture. By studying dosage compensation, we expect to discover how the expression state of an entire chromosome can be established and maintained. We have shown that dosage compensation is achieved in C. elegans by a complex of at least ten proteins that assembles on both hermaphrodite X chromosomes around the 40-cell stage of embryogenesis to reduce transcript levels by half. Although dosage compensation is a sex-specific process, the nematode dosage compensation complex contains both dosage compensation-specific proteins and general chromatin-associated proteins that are also active in meiosis or mitosis in both sexes. These proteins are similar to components of the mitotic chromosome condensation and segregation machinery (the 13S condensin complex) discovered in yeast and frogs, implying the evolutionary recruitment of ancient mitotic proteins to the regulation of gene expression. The multifunctional members of the dosage compensation complex are differentially commissioned for their roles in dosage compensation or chromosome segregation by their association with different protein partners. The protein complexes specialized for dosage compensation, mitosis, and meiosis are under analysis, along with their mechanisms of action.
Targeting the Dosage Compensation Complex to X Chromosomes. The dosage compensation complex (DCC) is recruited to X chromosomes by two hermaphrodite-specific genes (sdc-2 and sdc-3) that coordinately control both sex determination and dosage compensation. SDC-2 is central to X-chromosome recognition and confers both X-chromosome specificity and hermaphrodite-specificity to dosage compensation. The biochemistry underlying the function of SDC-2 is currently under investigation. While many components of the DCC have been identified, the cis-acting chromosomal elements that distinguish X chromosomes from autosomes to recruit the complex have been elusive until recently. To define these X-recognition elements (rex sites), we performed an X-chromosome-wide screen to identify regions of X sufficient to recruit the complex when detached from X. We found non-overlapping X regions that strongly recruit the DCC in vivo and other X regions that recruit the complex weakly or not at all, despite having genes know to be dosage compensated on the native X. The DCC normally binds the entire length of the wild-type X chromosome, including regions unable to recruit the complex when detached from X. Thus in worms, as in mammals, the DCC spreads into regions distant from initial recruitment sites. We have identified nine discrete X-recognition elements, and shown that nematodes use these cis-acting sites to recruit the DCC and nucleate spreading to neighboring stretches of X lacking recognition elements. This recruitment and spreading establishes the global, epigenetic regulation of X chromosomes that is maintained throughout the lifetime of the animal. Current projects define more precisely the mechanism of DCC spreading and the sequence or structure of rex sites responsible for differentiating X chromosomes from autosomes to recruit the DCC.
Regulation of Meiotic Crossover Interference in C. elegans. In most organisms, homologous chromosomes must undergo at least one crossover to guarantee proper segregation during meiosis. The number and distribution of crossovers is controlled through the process of crossover interference. In C. elegans, crossover interference is extremely tight, such that only one crossover event occurs per homologous chromosome pair per meiosis. Our recent experiments indicate that the dosage compensation protein DPY-28, a homolog of the 13S condensin subunit XCAP-D2, is important for this regulation of crossovers. Mutations in dpy-28 disrupt interference, causing double and triple crossovers per homolog pair and a skewing of the crossover distribution. The mechanism of crossover interference and its relationship to chromatin structure is under investigation.
Research Expertise and Interest
cell fate determination, chromosome architecture, developmental biology, gene expression, genetic determination of sex, regulatory genes, chromosome dynamics, X-chromosome
In the News
National Academy of Medicine elects three faculty members to its ranks
Genetic switch could be key to increased health and lifespan
Newly discovered genetic switches that increase lifespan and boost fitness in worms are also linked to increased lifespan in mammals, offering hope that drugs to flip these switches could improve human metabolic function and increase longevity.