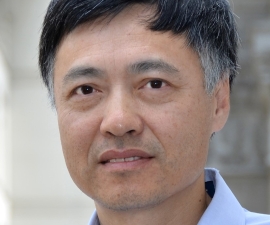
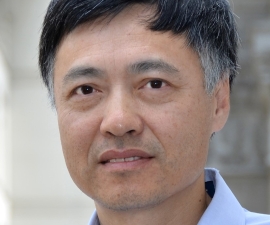
Research Bio
Electrons in solids determine most of the materials properties. An electron consists of charge and spin. While the electron charge determines the electronic properties, the alignment of electron spins generates magnetism. It is generally believed that a combination of the electron spin and charge freedoms in solids has a potential of creating new generation spintronics technology.
The removal of the spin degeneracy in magnetic materials leads to an inherent spin-charge correlation. Therefore magnetic nanostructures offer a great opportunity for tailoring the spin and charge properties at the nanometer scale. In fact, research on magnetic nanostructures has been developed rapidly in the last two decades with many exciting discoveries such as oscillatory interlayer coupling and giant magneto resistance (GMR).
Goal: To develop and realize the scientific and technological potentials of the magnetic nanostructures, it is important to understand: how do the electron spins behave at the nanometer scale? and how to create new types of nanostructures with desired properties? Answering these questions is the ultimate goal of the Qiu research group.
Research Expertise and Interest
experimental study of quantum magnetism in nanostructures, condensed matter physics, materials science experimentation