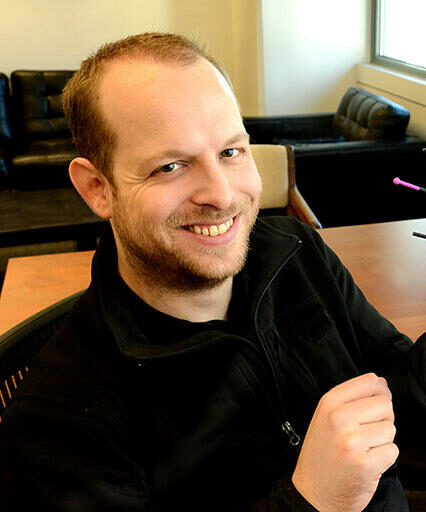
Felix Fischer
ChemistryFelix R. Fischer is an Assistant Professor in the Department of Chemistry at UC Berkeley. He is interested in the development of atomically unambiguously defined nanomaterials and their incorporation into functional electronic devices such as organic field-effect transistors, photovoltaic cells and integrated molecular circuits. Prior to joining the faculty at UC Berkeley, Felix was a German National Academy of Sciences Leopoldina Postdoctoral Research Fellow at Columbia University in New York.
Spark Award Project
Molecular Electronics and Sensing Based on Graphene Nanoribbons
The potential of single layer graphene based electronic devices has been recognized mostly due to its exceptionally high charge carrier mobility. While the extended two-dimensional hexagonal carbon lattice is a semimetal or a zero-gap semiconductor, structural confinement to an essentially one-dimensional graphene ribbon opens a band-gap large enough to expose room temperature semiconductor properties. The primary goal of Felix’s research is the development of a rational synthetic bottom-up approach towards atomically defined graphene nanoribbons that enables him to harness the exotic electronic and magnetic properties emerging from quantum confinement effects at the nanometer scale. His design of a new generation of high-performance electronic materials paves the way to meet the ever increasing demand for smaller, faster, and more energy efficient electronic devices.
Felix Fischer’s Story
“Ballistic transport ” – it sounds like a blast into the future
“Ballistic transport ” – it sounds like a blast into the future. And it is.
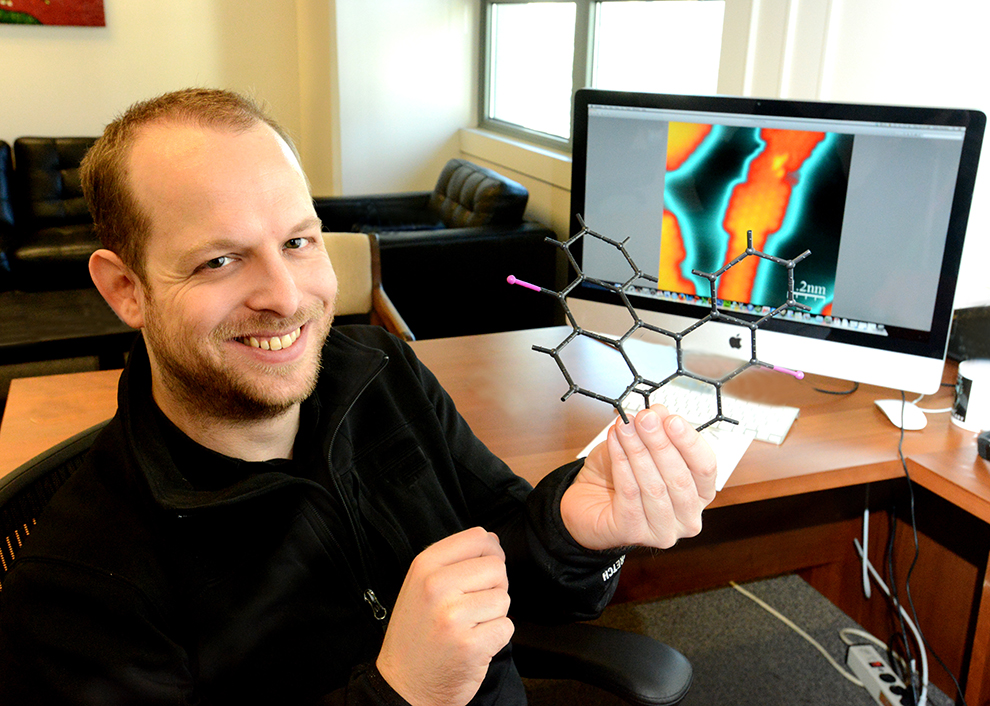
By fabricating strips of carbon only one-atom thick and less than 15 atoms wide, researchers aim to create molecular-scale “wires” capable of carrying information thousands of times faster than is possible today.
Crammed into integrated circuits, these microscopic strips known as graphene nanoribbons could increase by more than 10,000 times the number of transistors per area in computer chips. The exceptionally fast current transport along graphene nanoribbons would not only increase chip performance, but could refine the sensitivity of sensors to monitor circuit performance or subtle environmental changes.
First conceived only ten years ago, nanoribbon technology is, of course, a very hot field. To successfully exploit graphene’s great promise, though, the absolute dimensions of the nanoribbons and their internal symmetry must be precise and predictable. Variations in structure generate performance uncertainty and inefficiency. Today’s fabrication techniques aren’t yet up to the job.
Felix Fischer, a chemist and a member of the Kavli Energy NanoSciences Institute at Berkeley, is using his support from the Bakar Fellows Program to develop a totally new and extraordinarily precise way to create nanoribbons.
Fischer is also a recipient of a David and Lucille Packard Foundation Fellowship, awarded this year to 16 of the nation’s most innovative young scientists and engineers.
The conductivity and other electrical properties of nanoribbons are essentially defined by their dimensions. This, in turn, derives from their absolute atomic structure. Adding just one or two carbon atoms to a 15-atom-wide ribbon, for example, degrades its ability to work at room temperature.
Current fabrication methods rely on relatively crude physical means to create the microscopic strips – if something at the scale of less than a billionth of an inch can truly be called crude.
“The conventional approach uses a focused beam to carve nanoribbons from sheets of graphene,” Fischer says. “You chisel out the structure you want from a larger chunk of carbon. It can be done relatively quickly, but you don’t have precise control over the position of each carbon atom in the ribbon.
“We want nanoribbons in which we know exactly where each atom is.”
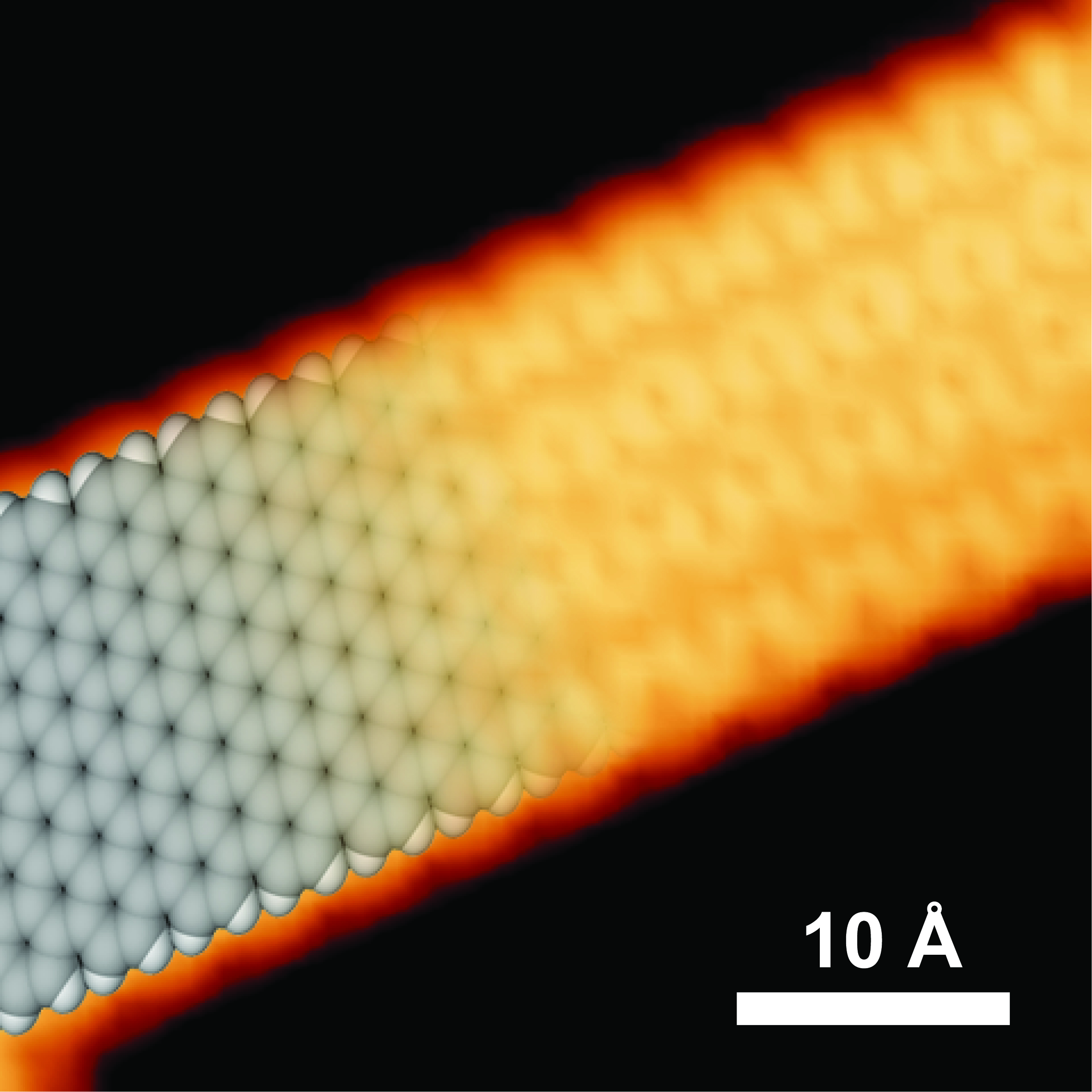
Instead of physically sculpting strips of graphene, Fischer chemically concocts them. By creating nanoribbons from their molecular subunits, he can control the position and number of each atom in the ribbon and achieve predictable control over their performance, he says.
His lab synthesizes molecular building blocks made from rings of carbon and hydrogen atoms, similar to the chemical structure of benzene. They then heat the molecules to link the building blocks into linear daisy-chains. In a second heating step the excess hydrogen atoms are stripped from the carbon skeleton yielding a uniform backbone of carbon-carbon bonds.
The assembly’s atomic arrangement and its supporting substrate look like snakeskin or a tire track – though at a phenomenally small scale. If 10,000 nanoribbons were placed side by side they would form a structure about as wide as a human hair.
Electrons can travel along the uniform graphene ribbon essentially with no atoms to block their way. Their straight trajectory enables them to transport current thousands of times faster over short distances than they would through a traditional metallic conductor like copper wire.
That, in turn, means that transistors can be switched on and off much faster – one of the keys to increasing a circuit’s speed.
Fischer has found that nanoribbons can operate as room temperature semiconductors when they are between 10 to 20 atoms wide.
“The wider the ribbon, the narrower the band gap (a determinant of electrical conductance),” he says. “If you go to much wider ones, the properties we need fizzle out.”
The graphene strips could enable much faster transport, storage, and retrieval of data than can today’s semiconductors. Their structure also dissipates heat well, which would allow computers and other large electronic devices circuits to work longer and more efficiently.
Leaning back in his chair, arms folded behind his head and a cheery smile on his face, Fischer likens his interest in nanoribbons to the excitement of a child dreaming of being an astronaut. “It’s being somewhere where no one has been before. In chemistry, you can make new things every day. You’re only limited by your imagination and creativity.”
He mentions the often-cited Moore’s Law that predicts the performance to computer chips to double every two years. “Many manufacturers have worried that we might be hitting a ceiling. You have to think of how you can produce electronic devices that can work faster without generating more heat. These nanoribbons might be a key to keeping up with Moore’s Law.”
Certainly imagining that possibility is the first step..